QUANTUM WELL : PICTURE
It can be seen that if the temperature is high(seldom encountered) or the applied field (VDS) is high, the electrons are excited to high energies and may escape from the triangular well. It may also be scattered into conduction band of the barrier(spatial transfer) and the carriers would be lost from the channel. To avoid this a quantum well may be introduced at the interface.
Actually in this case we work at several possibilities of hetrostructures.
Charge transfer occurs leading to a conducting channel within single quantum well or multiple quantum well. The energies of the electrons are quantized in the step like density of states. Therefore many carriers can be put in the channel with a narrower dispersion of energies.
Electron wave inside well is
This is Kane model.
Where x is the growth direction and k is the transverse electron wave vector.
block wave form and is the envelope wave function which is the solution of
Where is the effective mass, is the potential and is the confinement energy of carriers.
Boundary conditions are should be continuous at the interfaces. The triangular potential well is shown in Fig 17.1
The triangular quantum well Potential is linear for and at x = 0
The Schrödinger equation is
The boundary condition is
The above equation has two independent solutions.
One solution that is nonsingular at is AIRY function (Ai), so the resulting wave function is
as shown in Fig 17.2
The quantized energy levels are
Where, is the nth zero of Ai(x), hence
So
The basic idea of HFET or HEMT also known as MODFET- Modulation Doped Field Effect Transistor is that at equilibrium charge transfer occurs at the heterojunction to equalize the Fermi level on both side. Doping the N side gives wide base. Electrons are transferred to the GaAs side until an equilibrium is reached, this occurs because electron transfer raises the Fermi level on the GaAs side due to filling of the conduction band by electrons and also raises the electrostatic potential of the interface region because of the more numerous ionizer donors in the AlGaAs side. This charge transfer effect makes possible an old dream of semiconductor technologist, ie getting conducting electrons in a high mobility, High purity semiconductor without having to introduce mobility limiting donor impurities.
The various charge transfer mechanisms in heterojunctions are
- Electric charges and field near the interface determine the energy band bendings in the barrier and in conducting channel.
- The quantum calculation of the electron energy levels in the channel determines the confined conduction band levels
- The thermodynamic equilibrium conduction determine the density of transferred electrons.
Assuming that before the charge transfer the potential is flat band. After charge transfer of electrons the electric field in the potential well created can be taken as constant to first order and is given by
Gauss' Electrostatic potential is given by
Hence the Schrödinger equation for the electron envelope wave function is
The energy level in infinite triangular potential well for the ground state is
Where and is usually determined experimentally.
As charge transfer increases, potential created by transferred electrons also increases, leading to the lowering of the bottom of the Conduction . A 2DEG is formed when the Conduction band goes below the Fermi level, hence we get
and the energy in channel is given by 
In AlGaAs Fermi energy level is pushed downwards by electrostatic potential built up at the interface, where
Where W is the width of depletion region (Vdep ) in AlGaAs
Where is the donor on AlGaAs
Calculating energies from the bothom of Conduction Band, we get
and
Where is the donor binding energy in AlGaAs.
The donor concentration is equal to i.e, the number of electron transferred.
Hence we have
From the above equation NS can be calculated if the other parameters are known. The Fermi level is determined empirically by the model given by
In practice undoped AlGaAs spacer layer of thickness is used to separate Donor atom from channel electrons (2DEG) to prevent coloumb interactions resulting in an increased mobility is decreased as shown in Fig 17.2
The charge in the conduction band is
Where
We have calculated the 2DEG charge density N S and it can be related to the gate voltage by
with 
|
Where , is the 2DEG capacitance per unit area as given by
Where and is usually determined experimentally.
As charge transfer increases, potential created by transferred electrons also increases, leading to the lowering of the bottom of the Conduction . A 2DEG is formed when the Conduction band goes below the Fermi level, hence we get
and the energy in channel is given by 
In AlGaAs Fermi energy level is pushed downwards by electrostatic potential built up at the interface, where
Where W is the width of depletion region ( ) in AlGaAs
Where is the donor on AlGaAs
Calculating energies from the bothom of Conduction Band, we get
Where is the distance of the centroid of 2DEG from x = 0 as shown in Fig 17.3 and is usually
Again the threshold voltage or pinch off voltage is given by
Where is the Schottky barrier height on the donor layer as shown in Fig 17.4
At room temp also modulates the bound carrier density in Donor layer and free electron in Donor layer.
For a simplest model is so large that all electrons in 2DEG channel move with independent of as shown in Fig 17.5 and 
Where is the electron density per surface area and is given by , z is the gate width and is the gate length.
The transconductance is
 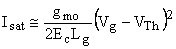  
So we have
Simplest model.
With we have and with we have 
The voltage in the channel and the current is given by
Hence current is given by
Where is the position of entrance to channel on the source side.
The saturation current is the current for which field on the drain side at just reaches is
Where, is the source resistance
For we get |
a linear behavior for a short highly conductive channel. The I-V characteristic is shown in Fig 17.6
for we get
|