FORMATION OF CARBON MONOXIDE
Carbon monoxide is formed during combustion of fuel-rich mixtures due to deficiency of oxygen. Combustion of hydrocarbon fuels may be considered as a two-step process leading to complete combustion when carbon dioxide is the final product.
Step 1
Conversion of hydrocarbons to CO: oxidation reactions involving intermediate species like smaller hydrocarbon molecules, aldehydes, ketones etc lead to formation of CO as schematically shown below are.
RH represents a hydrocarbon where R stands for the hydrocarbon radical
Step 2
Conversion of CO to CO2 : when sufficient oxygen is available. Hydroxyl radical OH is one the principal oxidizing species and converts CO to CO2,
The reaction (2.29) is quite fast and is under equilibrium at high temperatures. In fact, the reactions involving C-O-H system may be taken in chemical equilibrium during combustion and large part of expansion stroke when temperatures are above 1800 K.
CO emitted is higher than the equilibrium concentrations corresponding to the temperature and pressure conditions at the end of expansion. The calculations show that until about 60 degrees after top dead centre, the burned gases are close to equilibrium. However, late in the expansion stroke and during exhaust blow down on opening of the exhaust valve as the gases cool down, the CO concentrations differ from the equilibrium value. The predicted CO levels at the end of expansion computed by equilibrium considerations during early part of expansion and CO oxidation kinetics ( Reaction 2.29) in the later part of expansion correlated well with the experimental data as shown on Fig. 2.11 These CO values may be considered as partial equilibrium vales. Detailed investigations have shown:
- For rich mixtures (φ>1), the average exhaust CO concentrations are close to equilibrium concentrations during expansion.
- For near stoichiometric mixtures (φ ≈1) exhaust CO is close to computed partial equilibrium values.
- For lean mixtures the measured CO is higher than the computed values using kinetic models. This discrepancy may occur due to partial oxidation of unburned hydrocarbons released from crevices and lubricating oil film and deposits on the combustion chamber walls during expansion.
For estimation of CO concentration a good approximation is to assume chemical equilibrium frozen at 1750 K.
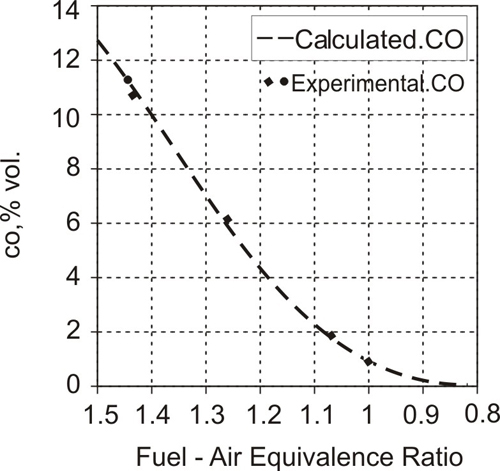
Fig 2.11 |
Comparison of calculated CO using partial equilibrium (kinetics in later part of expansion stroke) and experimental data |
CO emissions in real engines:
- Mixture mal-distribution in multicylinder engines causes cylinder-to-cylinder variation in air-fuel ratio. It results in significant increase in the average CO emissions. This is especially prominent in the carburetted or single point throttle body-injected (TBI) engines.
- Another contributing factor to higher CO emissions is non-uniform mixture distribution within the cylinder.
- During cold start of engine and acceleration rich mixtures are used resulting in higher CO emissions
Overall, the air-fuel ratio is the most important engine parameter affecting CO emissions. Other factors influence CO mostly indirectly through changes in mixture composition and/or promotion of slow oxidation reactions resulting in incomplete combustion.
|